The flow of genetic material between populations is a powerful force that shapes the biodiversity of our planet. Geneticists often think of its power to homogenize — to strengthen the connections and reduce the differences between groups — but gene flow is also creative. The introduction of new versions of genes (alleles) into a population by mating between species, or hybridization, can open up genetic avenues for adaptation. At its most extreme, gene flow can introduce factors that allow the resulting hybrid offspring to thrive independently of their parental taxa — becoming a new evolutionary lineage and, eventually, a separate species. Although hybridization has long been recognized as a key player in the formation of new species (speciation) when it coincides with a genome-doubling event (polyploidy), it is much rarer for hybrid species to form when genome-copy numbers are maintained (homoploidy). Writing in Nature, Rosser et al.1 provide evidence that hybridization has enabled the formation of a new homoploid lineage of longwing butterflies.
Read the paper: Hybrid speciation driven by multilocus introgression of ecological traits
Heliconius is a genus of brightly coloured butterflies that signal their toxicity through their wing patterns and other related traits (phenotypes). Two species — Heliconius elevatus and Heliconius pardalinus — are closely related but display striking differences in these traits. H. elevatus noticeably resembles its distant relative, Heliconius melpomene. Much of its colour pattern has been explained by the introduction of new alleles of two genes (cortex and optix) from H. melpomene into H. elevatus2–4. Although this work highlighted the adaptive role of gene flow in these butterflies, direct evidence that hybridization initiated the origin of H. elevatus has been missing.
Why are homoploid hybrid species so rare? Although it is now recognized that hybridization, and the resulting genetic exchange between species (introgression) is ubiquitous in nature5, showing that hybridization has created a new species requires an extra burden of proof. Biologists have argued that three criteria must be met before a hybrid lineage can be considered a species6. First, researchers must show a history of introgression. Rosser et al. provide a genome-wide description of introgression, indicating that less than 1% of the H. elevatus genome is derived from introgression from H. melpomene, and that the introgression event probably occurred around 180,000 years ago (Fig. 1).
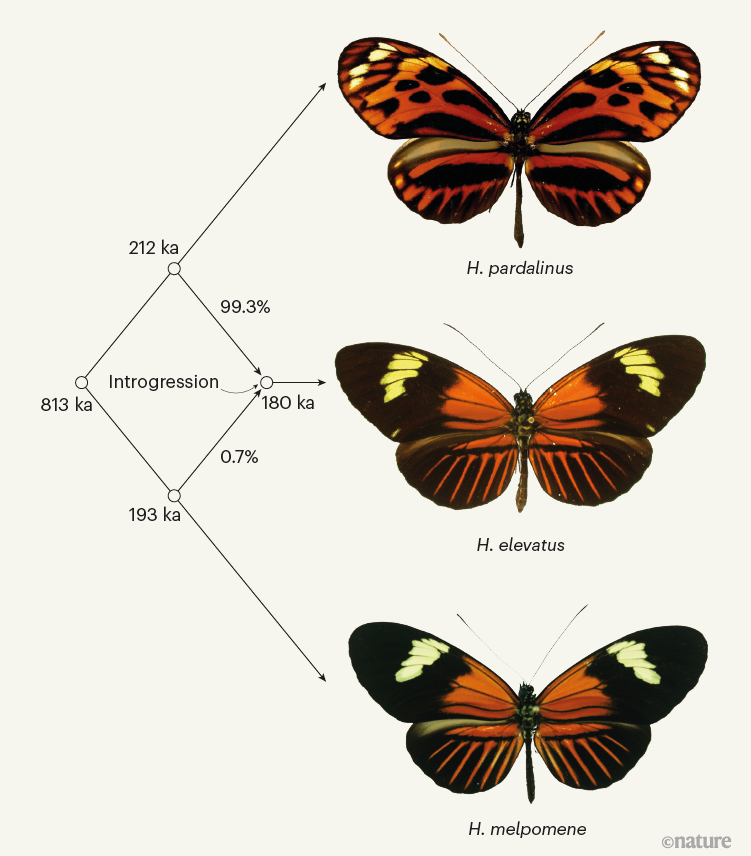
Figure 1 | The hybrid origins of a species of butterfly. The formation of a new species by hybridization (mating between two separate species), without changes to its genome-copy number, is relatively rare in nature. Rosser et al.1 outline a compelling case for such ‘homoploid’ hybrid speciation in Heliconius butterflies. The authors provide evidence that Heliconius elevatus is a hybrid between two distantly related parental species, Heliconius pardalinus and Heliconius melpomene. They find that only 0.7% of the H. elevatus genome is derived from H. melpomene, but these parts of the genome encode traits — including wing colouration and shape, male pheromones and flight dynamics — that facilitate the reproductive isolation of H. elevatus from H. pardalinus. The nodes indicate how many thousands of years ago (ka) the three species and their common ancestor are thought to have originated, and when genetic exchange (introgression) between H. pardalinus and H. melpomene is estimated to have occurred.
However, simply demonstrating a history of introgression is not enough. In the era of genomic data, there has been no shortage of evidence for gene flow in the history of species from all parts of the tree of life, including in butterflies5,7. Second, researchers must also demonstrate that reproduction between the putative species and its parental taxa is impeded (that is, they are reproductively isolated). For two species to be considered freely interbreeding, they must be able to meet, mate and produce viable and fertile offspring. Barriers to reproduction can occur at any point in this process. Earlier studies have found evidence that H. elevatus prefers to mate with individuals of its own species8, rather than with individuals of H. pardalinus — a type of reproductive isolation known as assortative mating. Furthermore, there is evidence that, in at least some populations, hybrid female offspring from the two species cannot produce offspring of their own — another type of reproductive isolation known as hybrid sterility8.
Cichlid fish seized an ecological opportunity to diversify
Rosser et al. further demonstrate that H. elevatus is strongly reproductively isolated from H. melpomene, but more weakly isolated from H. pardalinus. Nonetheless, despite a clear history of gene flow between H. elevatus and H. pardalinus, the two species form distinct genetic groups, indicative of the maintenance of species boundaries in the face of high gene flow.
The third and final criterion for being considered a hybrid species is the hardest to meet and happens most rarely: researchers must show evidence that gene flow has directly led to reproductive isolation. Rosser et al. use a technique called quantitative trait locus mapping to relate genetic variants at different regions (loci) of the genome with variation in numerous traits that are associated with assortative mating, such as the colour and shape of the butterfly’s wings, its preference of male mate and its preferred plant on which to lay eggs. They find a striking alignment between the loci underlying key differences between H. elevatus and H. pardalinus and regions of introgression from H. melpomene. This monumental mapping effort provides powerful evidence for the direct role of hybridization in the origin of barriers to reproduction.
Remarkably, despite the history of gene flow between H. elevatus and H. pardalinus, the distinction between the two species, or differentiation, is maintained at several key loci. Importantly, these loci are found throughout the genome, and cannot be entirely explained by pleiotropy (in which a single locus affects multiple traits) or inversions (through which matching alleles at different loci become physically locked together). In 2023, a similar pattern to this was uncovered in Penstemon, a wildflower that exhibits multiple evolutions of hummingbird-pollinated flowers from closely related bee-pollinated species9. As with the butterflies, the two groups are distinguished by a suite of traits that always appear together. These phenotypes are controlled by numerous independent ‘barrier’ loci found across the genome, but gene flow is rampant outside these distinct regions.
Deadly mismatch between nuclear and mitochondrial genes in swordtail fish hybrids
It is often theorized that the build-up of many differences across the genome should lead to differentiation between species as the effects of the barrier loci spread to neighbouring neutral loci10, but for both Heliconius and Penstemon, there is no evidence that loci underlying species differences prevent gene flow at nearby neutral loci. Crucially, the dynamics of ongoing gene flow can be stable, persisting for hundreds of thousands of generations1. Studies such as these can reshape scientists’ thinking about what is required to maintain species distinctions in the face of high gene flow.
The story of H. elevatus is not only the story of a rare, homoploid hybrid speciation event, but one of the complex nature of gene flow itself. Gene flow can be both a homogenizing and a diversifying force in evolution. Researchers will require both broad views of genome-wide patterns and detailed information about the genetic basis of multiple phenotypes to fully understand the relationship between genomic and phenotypic evolution. Scientists are still just beginning to disentangle the complex histories that have produced modern biodiversity, and studies such as that by Rosser et al., which combine large-scale genomics with genetic mapping for multiple phenotypes, provide a clear path forwards in our developing understanding of speciation.